This article addresses the growing concerns of passive intermodulation (PIM) and other forms of interference in 5G networks. It explores the unique challenges introduced by new frequency bands and highlights effective strategies for troubleshooting these issues. The article reviews current industry trends in PIM detection and discusses innovative solutions tailored for RF engineers.
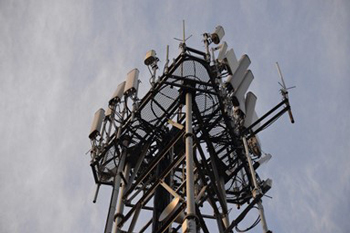
Figure 1 Tower-mounted antennas.
The advent of 5G technology represents a major evolution in telecommunications. It is characterized by unprecedented data speeds, vastly reduced latency and increased capacity capable of supporting an immense number of connected devices simultaneously. However, the robust deployment and performance of 5G networks are significantly challenged by PIM and various interference issues, which can severely degrade network quality. Figure 1 shows an example of a tower used by wireless networks. It is easy to see how multiple antennas and devices communicating with these antennas can create interference.
PIM occurs when multiple signal frequencies interfere with non-linear passive components like antennas and cables. These frequencies can generate spurious signals that interfere with the receiver's ability to decode the desired signals. Furthermore, frequency allocation plays a crucial role; ensuring that signal transmissions are carefully planned to avoid frequencies that exacerbate PIM effects is vital.
The 5G network operates in dense signal environments and higher frequency spectrums. Alongside PIM, other electronic devices can disrupt these networks, and the colocation and lack of synchronization in TDD networks can exacerbate these challenges. The potential consequences of PIM and interference are significant, including increased call drops, reduced data throughput and diminished overall network capacity.
Effective management of PIM and interference is essential for maintaining the performance integrity of 5G networks. It requires meticulous engineering to reduce PIM sources through improved component design, installation precision and ongoing network maintenance. Addressing PIM and interference effectively enhances network performance reliability and expands the range of capabilities. This allows these networks to be the cornerstone for many applications ranging from IoT to ultra-reliable low-latency communications crucial for autonomous vehicles and advanced industrial automation.
5G NETWORKS
Unlike previous generations, 5G networks encompass a wide range of frequencies and utilize advanced technologies to achieve superior network efficiency and performance. 5G networks operate across three primary frequency bands, each offering distinct benefits for different use cases:
Low-Band (< 1 GHz): This spectrum includes frequencies like 600 MHz, 700 MHz and 850 MHz. Known for its extensive coverage and deep indoor penetration, the low-band spectrum is crucial for broad, regional coverage, including in rural areas. While it ensures excellent coverage and connectivity, the low-band spectrum offers relatively lower data speeds and limited bandwidth capacity, which may not support the high-speed requirements expected in dense urban settings.
Mid-Band (1 to 6 GHz): Mid-band spectrum, which includes AWS, PCS, WCS, 2.3 GHz, 2.5 GHz, CBRS (3.5 GHz) and 3.7 to 4.2 GHz frequency bands, strikes a balance between coverage and capacity. It offers faster speeds and lower latency than low-band frequencies, making it ideal for urban and suburban areas. The mid-band spectrum is extensively used for general mobile connectivity and efficiently handles higher data demands.
High-Band or Millimeter Wave (24 GHz and above): High-band spectrum encompasses frequencies like 28 GHz, 37 GHz and 39 GHz. It can support extremely high data speeds and ultra-low latency. It is suitable for high-demand applications requiring massive real-time data transmission, such as virtual reality or live streaming in high-definition. However, due to its shorter range and higher susceptibility to physical obstructions, the high-band spectrum requires densely deployed small cells to ensure effective coverage.
TECHNICAL SPECIFICATIONS
- Bandwidth: Available 5G bandwidth varies significantly across different spectrum bands:
- Low-Band: Approximately 10 to 20 MHz per channel
- Mid-Band: Typically, 50 to 100 MHz per channel
- High-Band: From 400 to 800 MHz or more.
- Output Power: Power levels also vary with frequency bands:
- Low-Band: Generally, around 40 to 60 W per channel to cover larger areas
- Mid-Band: Typically, 20 to 30 W per channel
- High-Band: Around 1 to 3 W per channel due to the short-range nature of the transmissions.
- Modulation and Duplexing Techniques:
- Modulation Techniques: 5G NR uses modulation techniques like QPSK, 16-QAM, 64-QAM and 256-QAM to maximize spectrum efficiency
-
Duplexing Schemes:
- FDD: Predominantly used in lower frequency bands, suitable for stable, continuous communication.
- TDD: Commonly used in mid to high-frequency bands, offering flexibility to manage asymmetric data flows and adapt dynamically to varying traffic conditions.
5G technology has set new benchmarks in telecommunications with its high data rates, increased capacity and significantly reduced latency. However, these network enhancements bring complex interference challenges, particularly PIM, which can severely impact the network’s efficiency and reliability. Understanding and mitigating these interference mechanisms is crucial for maintaining optimal network performance. Although 5G networks are deployed in various frequency bands, this article focuses on interference and PIM mitigation in C-Band (3700 to 3980 MHz), CBRS (3550 to 3700 MHz), DoD (3450 to 3550 MHz), ESR (4000 to 4200 MHz) AND FAA (4200 to 4400 MHz) frequency ranges.
WHAT IS PIM?
PIM occurs when two or more signals mix non-linearly in passive components like antennas, cables or connectors to produce unwanted spurious signals known as passive intermodulation products. These products are typically designated as IM2 (second order), IM3 (third order), IM5 (fifth order), etc. IM3 is often the most problematic, primarily because this product is likely to fall in the receive band in FDD networks and can be powerful enough to interfere significantly with the receiver's ability to decode the intended signals. An example of two signals closely spaced in the downlink and how the mixing products can interfere with the desired signals is shown in Figure 2.
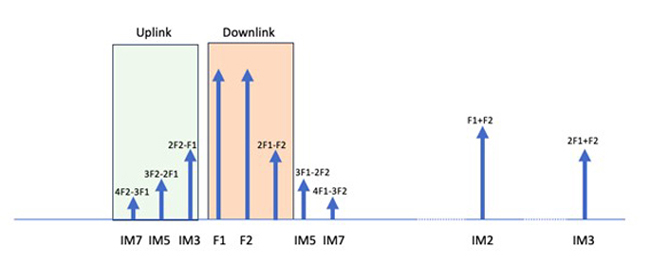
Figure 2 Intermodulation products from two closely spaced signals.
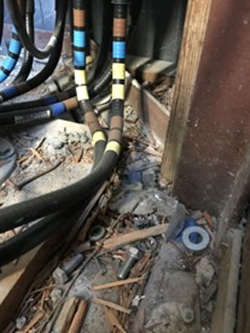
Figure 3a Loose metal parts.
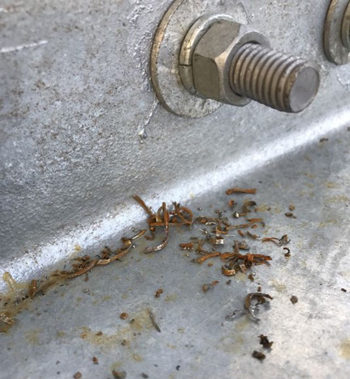
Figure 3b Metal swarf.