
Parabolic-reflector antennas are widely used as microwave directional antennas. Unfortunately, the illumination of a parabolic mirror becomes rather inefficient when the dimensions of the mirror are not large enough compared to the wavelength. On the other hand, the directional gain of microwave cavity antennas1 is rather small, since it is not easy to build a short-backfire antenna (SBFA)2 with a directivity larger than approximately 17 dBi.
An antenna with a required directivity in the range between 19 and 22 dBi is too large a value for a single SBFA. On the other hand, this value is too small to efficiently illuminate a parabolic reflector. The archery-target antenna described in this article and shown in Figure 1 is an attempt to upgrade the SBFA to obtain a higher directivity and/or more gain.
Increasing the directivity requires a larger aperture. If a SBFA is made too large, its directivity will decrease due to the increasing phase and magnitude errors of the aperture illumination. In the archery-target antenna, the phase of the aperture illumination is further controlled by an additional annular reflector, installed in the same plane as the small reflector of a traditional SBFA.

Fig. 1 The archire-target antenna.
The design of the archery-target antenna is shown in Figure 2. The additional annular reflector shades part of the aperture, so that the aperture radiates from two annular sections: between the small and annular reflectors, and between the annular and large reflectors. The size of the annular reflector has to be adjusted so that both annular apertures are fed in phase. One can expect, of course, that the illumination efficiency will be somewhat compromised due to the shading of the annular reflector.

Fig. 2 Design of the archire-target antenna.
The approximate dimensions of the archery-target antenna, obtained from many radiation-pattern measurements on different prototypes, are shown in Figure 3. The large reflector has a diameter of approximately five wavelengths and is much larger than in a typical SBFA. On the other hand, the small reflector has a diameter of 0.7 wavelength and is comparable to the SBFA. The annular reflector extends from an inner diameter of 2.2 wavelengths to an outer diameter of 3.7 wavelengths. The reflector spacing and rim height are identical and equal to 0.7 wavelength. This figure is also somewhat larger than in a typical SBFA.

Fig. 3 Dimensions of the archery-targert antenna.
A possible explanation of the operation of the archery-target antenna is that the inner annular aperture between the small and annular reflector operates in the same way as the SBFA. The annular reflector width (about 0.75 wavelength) has to be adjusted to feed the outer annular aperture between the annular and large reflectors with the correct phase. In particular, the parallel-plate waveguide between the annular and large reflectors should introduce a phase shift of 180°.
Considering the radiation pattern of the dipole feed, the parallel-plate waveguide between the annular and large reflectors is mainly excited by the lowest order TE mode. If the reflector (plate) spacing is reduced to less than 0.7 wavelength, the phase velocity of the TE mode increases. This requires a much wider annular reflector, the shading increases and the overall aperture illumination efficiency is compromised.
Measured Antenna Performance
All of the results presented in this article were obtained from several practical radiation-pattern measurements on many antenna prototypes. Due to the limitations of the available test equipment and antenna test range, all of the measurements were performed at S-band frequencies in the range between 2.2 and 3 GHz. In this frequency range, the described antenna has the size, shape and appearance of an archery target.
The best prototype antenna has a large reflector diameter of 570 mm, an annular reflector with an inner diameter of 252 mm, an outer diameter of 420 mm and a small reflector with a diameter of 80 mm. The reflector spacing and rim height are set to 80 mm. The small and annular reflectors are carried on a large dielectric plate made of 0.8 mm thick glass fiber-epoxy laminate with a dielectric constant of approximately 4.5. Although thin, this carrier plate has the effect of decreasing the optimum frequency by as much as 100 MHz in the S-band frequency range.
The prototype antenna achieved the best directivity of 20.6 dBi at an operating frequency of 2640 MHz. This directivity was computed from the measured E- and H-plane radiation patterns shown in Figures 4 and 5. The measured E-plane –3 dB beam width at 2640 MHz is 13.8°, while the measured H-plane –3 dB beam width at 2640 MHz is 10.2°.

Fig. 4 Measured E-plane radiation pattern (40 dB scale).

Fig. 5 Measured H-plane radiation pattern (40 dB scale).
Both E- and H-plane radiation patterns have many relatively weak side lobes between –13 and –17 dB due to the shading of the annular reflector. The side lobes are well visible in the figures, due to the 40 dB logarithmic scale. When the operating frequency is increased, the main lobe becomes narrower and the levels of the side lobes increase.
The directivity obtained from the measured E and H radiation patterns between 2400 and 2800 MHz is shown in Figure 6. The curve shows that the archery-target antenna is capable of operating over a frequency range of 10 to 15 percent of the center design frequency with just a small (0.5 to 1 dB) performance degradation. The accuracy of the antenna test range used for the pattern measurements is estimated to be ±0.2 dBi for directivity determination.

Fig. 6 Directivity from the measurede E and H patterns.
Illumination Dipole Design
The first experiments with the archery-target antenna were made with a simple thin-wire, half-wave, dipole feed. The dipole was positioned on the antenna axis of symmetry exactly halfway between the small and large reflectors just like in a SBFA. Since the thin-wire dipole had a poor impedance match to a 50 ? source, even over a narrow frequency band, due to the antenna cavity loading, several other feeds were experimented, as shown in Figure 7.

Fig. 7 Different feeds for the archery-target antenna.
A reasonable impedance matching (15 dB return loss over a 10 percent bandwidth) was obtained with a single wide-dipole feed, built on a printed-circuit board, as shown in Figure 8. While experimenting with different feeds, small (up to ±0.2 dBi) but repeatable variations of the antenna directivity were also observed. In particular, the directivity decreased when the wide-dipole printed-circuit board was installed parallel to the reflector plates.

Fig. 8 Wide-dipole feed for the archery-target antenna.
On the other hand, the directivity improved when the wide-dipole printed-circuit board was installed perpendicular to the reflector plates. The feed radiation pattern can therefore contribute to a more uniform illumination of both annular apertures of the archery-target antenna. Similar results were also obtained with a double dipole feed, as shown in Figure 9, including directivity improvements and impedance matching.

Fig. 9 Double-dipole feed for the archery-target antenna.
All the data presented in this article were obtained with the double dipole feed. The approximate dimensions of the two best-performing feeds are shown in Figure 10.
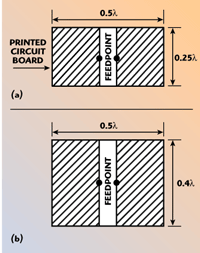
Fig. 10 Design dimensions of the (a) single-wide and (b) double illumination dipoles.
Mechanical Design of a Weatherproof Antenna
Photos of an early prototype of the archery-target antenna are shown in Figures 11 and 12. The large reflector and the rim are made of aluminum sheets, while the small and annular reflectors are carried by an insulating plate (0.8 mm thick glass fiber-epoxy laminate). The insulating carrier plate extends over the entire aperture of the antenna, so the dipole feed is not visible.

Fig. 11 Front view of the prototype archery-target antenna.

Fig. 12 Rear view of the prototype archery-target antenna.
The mechanical design of a weatherproof antenna is shown in Figure 13. The antenna includes only three components: a metal cup serving as the large reflector, a printed-circuit board carrying the small and annular reflectors while simultaneously serving as a radome, and the dipole feed. When used for terrestrial links, the radiating surface is vertical, so moisture, snow and even ice will hardly accumulate on the radome.

Fig. 13 Mechanical design of the weatherproof antenna.
Conclusion
A new type of microwave cavity antenna is presented in this article, achieving a relatively high directivity of 20.6 dBi with a –1 dB directivity bandwidth of approximately 15 percent of the center frequency. A partial disadvantage is that the aperture efficiency only reaches 46 percent. On the other hand, the antenna requires few mechanical parts, is simple to manufacture and the radome is integrated in the antenna. Except for the feed, the whole antenna design is polarization-independent. Therefore, only the feed needs to be changed for a different and/or dual polarization.
At least three possible improvements of the archery-target antenna were not yet tested. First, more than one annular reflector could be used to further increase the aperture area and directivity. Second, the space between the annular and large reflectors could be filled with a natural or artificial dielectric to decrease the TE-mode phase velocity, reduce the annular reflector width, reduce the shading and improve the aperture efficiency. Third, several other feeds could be tried to obtain a more uniform aperture illumination. n
Acknowledgments
The author would like to thank Mr. Stanko Gajsek for the accurate measurements of the many different prototypes of the archery-target antenna. The author would also like to thank Prof. Ahmed Kishk for the accurate computer simulations and for his many useful suggestions in writing this article.
References
1. H.D. Hristov Kumar, Microwave Cavity Antennas, Artech House Inc., Norwood, MA, 1989.
2. H.W. Ehrenspeck, “A New Class of Medium-size, High Efficiency Reflector Antennas,” IEEE Transactions on Antennas and Propagation, March 1974, pp. 329–332.