WHAT IS RFOF?
As currently deployed and widely used in many market segments, RFoF is a technology that transfers electromagnetic signals over fiber-optic links via amplitude modulation. A basic link consists of a transmitter at one end, composed of a semiconductor laser and a matching circuit that controls the light intensity according to an input signal and an optical receiver at the other end. The receiver is a photodetector aligned with an optical fiber and a circuit that amplifies the signal and drives the output. Fiber-optic technology is widely used for the Ethernet backbone and all digital data networks. The same fibers that inherently have very low optical losses transport the light modulated by the transmitter over a significant range, often at distances up to tens of kilometers. The modern RFoF systems are modular and offer significant signal grooming/matching and control for higher frequencies. Transport at 40 GHz is routine and transport systems operating at 60 GHz have been demonstrated. A simplified block diagram for RFoF network transmission is shown in Figure 2.

Figure 2 Bi-directional RF signal transport over fiber.
The scalability and adaptability of RFoF systems contribute to their efficacy in evolving technological landscapes. These systems can be easily integrated into existing infrastructure, offering seamless upgrades and expansions without the need for extensive overhauls. The modular nature of RFoF allows for the incorporation of advanced features and functionalities, enhancing signal processing, minimizing latency and optimizing performance. This adaptability is important for telecommunications and big data sectors, where the demand for higher bandwidths and improved signal integrity continues to grow. Despite how vital this technology has become for many industries; it comes from humble beginnings.
BRIEF HISTORY
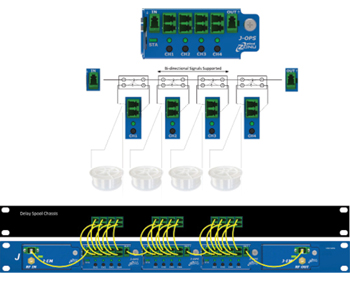
Figure 3 Programmable RFoF time delay system for radar simulation and testing.
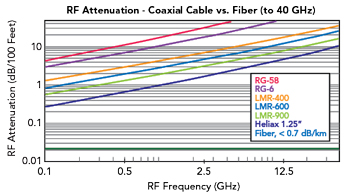
Figure 4 RF attenuation to 40 GHz.
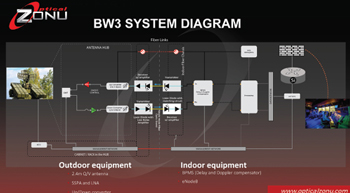
Figure 5 Antenna remoting with RFoF link.

Figure 6 RFoF components.
RFoF emerged during the second generation of CATV distribution, a period when TV channels were transmitted through analog modulation across the nation. In the 1990s, the original equipment, initially operating up to 800 MHz and later expanding to 1 GHz, utilized RFoF technology to deliver video signals. These signals were transmitted to a node and then distributed to individual subscribers’ homes via coaxial cables.
During this era, CATV served as the primary market application for RFoF. As the demand for VHF transport increased, RFoF found applications in various niche sectors. RFoF technology began to play a crucial role in satellite communication, ground stations, telemetry, audio/video distribution in studios and impromptu events. The technology also contributed to enhancing cellular network coverage in key locations such as airports, stadiums and campuses.
RFoF applications also extended to unique instrumentation scenarios, where programmable time delay became widely adopted for radar testing. This adaptability showcased the capability of the technology to meet the specific needs of various industries and settings. Figure 3 shows a programmable RFoF time delay system for radar simulation and testing.
As technology advances, RFoF continues to evolve, with improvements in bandwidth, reliability and efficiency. The 1990s laid the foundation, but subsequent decades witnessed the refinement and expansion of RFoF applications, making it an integral part of modern telecommunications and broadcasting infrastructure. The journey from its inception in CATV applications to becoming a crucial component in diverse sectors exemplifies RFoF’s capacity to evolve and address the ever-changing demands of the telecommunications landscape.
WHY RFOF IS BECOMING INCREASINGLY IMPORTANT TO THE MILITARY
For military applications, RFoF plays a vital role as a replacement for coaxial cables or waveguides, enhancing the efficiency and versatility of communication systems. RFoF uses analog signals to modulate the light intensity of specific wavelengths, effectively converting electrical RF signals into an optical format. The signal then traverses a single-mode fiber for resilient and efficient low loss transmission and upon reaching its destination, it is converted back into an RF output to enable precise control and utilization. A significant feature of RFoF transmission is its ability to carry high dynamic range broadband signals over a single link and multiplex multiple wavelengths over a single strand of fiber for high density transport.
Fiber-optic cables have minimal signal loss over long distances, ensuring that data can be transmitted reliably, even across extended military networks. Coaxial cables, in contrast, are more susceptible to signal degradation over distance, necessitating signal boosters or repeaters. The difference in RF attenuation for coaxial cables and fiber is illustrated in Figure 4.
In addition to higher loss, coaxial cables are susceptible to electromagnetic interference that can disrupt or degrade signal quality. Fiber is also inherently more secure than coaxial cables since it does not emit electromagnetic radiation that can be easily intercepted. This feature makes the transport method resistant to electronic eavesdropping and signal interception by adversaries. This is why the military uses fiber for antenna remoting to separate mission-critical systems from its communication transmitter/receiver. RFoF will be a critical element of all military communications as electronic warfare becomes a growing and increasingly sophisticated issue.
There are a significant number of military-focused use cases for antenna remoting with RFoF. Figure 5 shows a simplified functional block diagram for what takes place between the control center and the remote antenna. Many applications are making more use of antenna remoting:
- Satellite Communication: Military satellite communication systems rely on remote antenna installations to establish and maintain connections with satellites in geostationary or low earth orbit. These antennas are often placed on ships, aircraft or in remote locations to ensure a reliable link with satellites.
- Radar Systems: Military radar systems, including ground-based, naval and airborne radar utilize phased array antenna technology. Control of signal phase and delay over fiber provides an advantage over coax cables or waveguides. Fiber-based radar simulators are used for calibration and training purposes.
- Covert Surveillance: Remote antenna systems can be camouflaged or hidden to conduct covert surveillance and intelligence gathering operations. These antennas are placed in concealed locations to avoid detection by adversaries.
- Secure Wi-Fi: Antenna remoting enables secure Wi-Fi in sensitive compartmented information facilities (SCIFs) by physically separating the external antennas from the secure facility. Fiber-optic connections are used to transmit signals and implement a controlled and secure internal Wi-Fi network. This approach prevents unauthorized access and eavesdropping while providing secure and reliable Wi-Fi access to authorized personnel within the SCIF. The same platform can also be used for full spectrum monitoring of the area covered by the Wi-Fi service.
It is important to note that the electronics in RFoF systems do not look substantially different than what would be expected in legacy systems. Only the transport medium looks different. Figure 6 shows RFoF components for a variety of system functions. Figure 7 shows the sub-system implementations for RFoF networks.
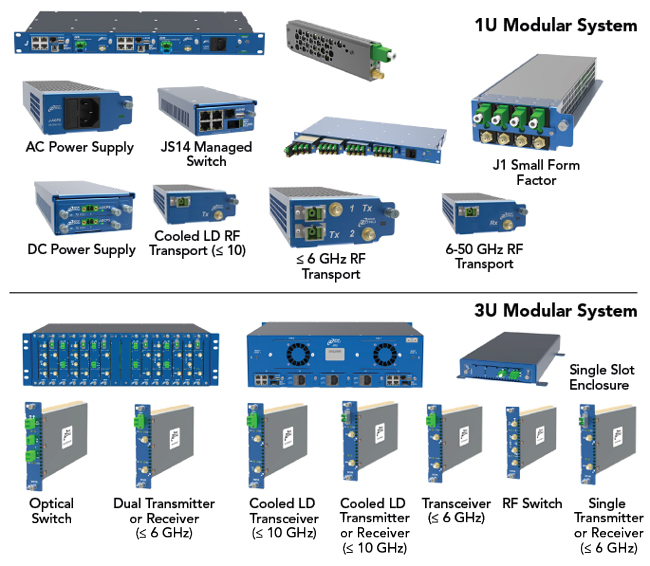
Figure 7 RFoF subsystems.
Fiber is also lighter weight with a smaller form factor. In military communications, size and weight matter, particularly in airborne applications like aerostats and drones. The size and weight of fiber cables make them easier to transport and install in military operations. They are also well-suited for field deployments where mobility and rapid setup are critical. Beyond size and weight, fiber is more durable than coaxial cable. It is less susceptible to environmental factors such as moisture, temperature fluctuations and corrosion.
U.S. NAVY CASE EXAMPLE
While using RFoF for autonomous military assets may feel like futuristic, many of these problems are being discovered and solved today. A compelling case study of the application of RFoF technology on autonomous vehicles for the military is exemplified by the U.S. Navy’s utilization of unmanned surface vessels (USVs). Ku-Band Common Data Link (CDL) communications payloads are routinely transported between radomes mounted high above USV decks and equipment rooms nestled within the ship’s hulls via antenna remoting.
Currently, waveguide and coaxial cables are used for such tasks, but they present significant RF losses and logistical difficulties. Coaxial cables are inherently inflexible and highly susceptible to mechanical stress like shock or vibration. Their fragility necessitates meticulous maintenance, a particularly daunting task during prolonged deployments at sea or in oceanic conditions. Service disruptions require extra time and resources to repair and may even create the need for the ship to go back to port for repairs.
Even minimal moisture or minute traces of dirt or salt can detrimentally impact waveform transmission and subsequently degrade RF performance. To mitigate moisture-related issues, waveguides are often filled with dried air or purified nitrogen, but any corrosion or oxidation of the inner metal surfaces further compromises their performance. In one instance, water entered the hull even with the nitrogen present and disrupted the RF signal.
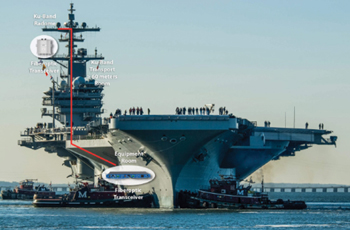
Figure 8 Antenna remoting use case.
To counter this issue, the Optical Zonu team worked with the U.S. Navy to successfully deploy fiber cables as a replacement for waveguides to fix the lingering issues. Fiber-optic technology demonstrated several key advantages over waveguides, including superior long-term performance and lower overall lifetime costs. The technology also proved to be much more flexible and easier to install with a bend radius of less than 1 in. Figure 8 shows the RFoF fiber run and location for the aircraft carrier.
The fiber-optic transport links proved capable of meeting and exceeding CDL requirements. They offered low loss transport, support for all CDL-compliant waveforms, symmetric/asymmetric data rates and comprehensive audio/video transport. These fiber-optic links enable Ku-Band RF transport over much longer distances than could be supported by waveguide technology.
CONCLUSION
The rapid expansion of autonomous vehicles and weapons within the military, driven by substantial funding from the DOD, represents a pivotal step in maintaining the U.S.’ military edge. However, this transformative shift is not without its challenges, chief among them being the resilience of the communication infrastructure that underpins these innovative systems. As unmanned military assets become increasingly integral to operations, their reliance on continuous, real-time communication, alongside advanced sensor technologies, becomes paramount. The potential for communication failures or adversarial detection poses a direct threat to operational integrity. Compounding these challenges is the vulnerability of higher frequencies, which support the low latency, high bandwidth communication networks essential for automation.
RFoF is emerging as a critical solution to safeguard and enable autonomous military systems. RFoF technology not only offers enhanced security and resilience but also addresses the evolving demands of modern warfare, ensuring that the U.S. can maintain its military capabilities in an increasingly complex and connected world. As the landscape of military technology continues to evolve, the strength of our communication infrastructure becomes a decisive factor in the success and reliability of our national defense systems.
Reference
- “Pentagon Gets $7.5 Billion for Unmanned Systems,” National Defense Magazine, May 2021, Web: nationaldefensemagazine.org/articles/2021/5/27/pentagon-gets-$7-5-billion-for-unmanned-systems.