New aerospace and defense systems must implement high performance radio equipment to communicate, operate and keep their assets safe. The battlefield is dynamic, so being able to adapt easily is extremely important. Software-defined radios (SDRs) are a type of RF transceiver that implements most of the radio operations through software. These radios can be reprogrammed to comply with different requirements and modify functionality. These devices are extremely flexible and versatile to help defense organizations maintain superiority. High performance RF devices are an integral part of aerospace and defense systems. For instance, radars are useful to detect and track physical targets in all domains, electronic warfare (EW) systems are critical tools to disrupt enemy communications and networks and spectrum monitoring and recording systems can intercept and analyze threats present in the electromagnetic spectrum. RF systems are also crucial for satellite communications, surveillance and navigation.
This article will compare high performance commercial off-the-shelf (COTS) SDRs for aerospace and defense applications, investigating their performance specifications in detail. SDRs are extremely popular in the aerospace and defense industry, as they provide flexibility, accuracy and robustness in a small and modular footprint. The article will cover the main requirements for different aerospace and defense applications, including radars, spectrum monitoring systems, EW devices, satellite communications, surveillance and navigation. It will also compare COTS SDR models from three suppliers: the Cyan model from Per Vices, the USRP X410 from National Instruments (NI) and the HTLw SDR from Herrick Labs. The analysis will investigate important specifications like frequency range, bandwidth, sampling rate, dynamic range, modulation schemes and signal-to-noise ratio (SNR).
WHAT IS AN SDR?
SDRs are an RF device that implements most of its radio functions in the digital domain. They can be easily modified to work with a wide range of applications through simple software updates, without requiring hardware changes. The core of an SDR is the radio front-end (RFE) and the digital backend, which communicate with each other through analog-to-digital (ADC) converters and digital-to-analog (DAC) converters. The RFE implements all the analog functions required for receive (Rx) and transmit (Tx) operations, including filtering, amplifying and mixing. The RFE specifications limit SDR performance, so the highest bandwidth SDRs in the market implement RFEs with DC to 18 GHz tuning ranges, which may be upgradable to 40 GHz. These RFEs typically have 3 GHz of instantaneous bandwidth, while also providing excellent SNR and dynamic range. MIMO SDRs are state-of-the-art in terms of RF functionality, implementing RFEs with multiple Rx and Tx channels that can work in parallel through independent ADC/DAC channels. The digital backend of an SDR typically contains a field-programmable gate array (FPGA) with onboard digital-signal processing capabilities for implementation of the basic RF operations. These operations include modulation and demodulation, up- and down-converting, data packaging and filtering. They also extend to complex radio protocols, smart algorithms and high throughput host communication interfaces. High performance SDRs also provide powerful host interfaces using qSFP+ ports for optical Ethernet links that can reach 400 Gbps performance, typically with four 100 Gbps lanes. Figure 1 provides a high level overview of SDR boards.
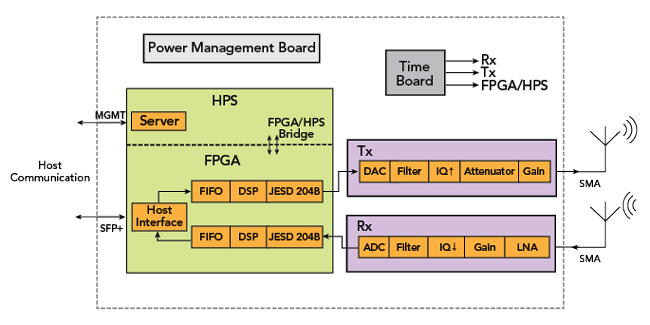
Figure 1 High-level view of an SDR.
SDR USAGE IN DIFFERENT APPLICATIONS
Radar systems are important in both aerospace and military defense applications. Radars come in many varieties: Doppler radars, synthetic aperture radars, ground-penetrating radars, pulse radars and continuous-wave radars. These systems are used for a variety of functions, like tracking, target identification, surveillance and imaging, so the RF transceiver must be able to adapt to a variety of conditions and specifications. One of the main advantages of SDRs in radars is the flexibility to configure the signal processing chain to adapt to changing operational requirements. This allows the system to adapt to jamming signals, challenging weather conditions and clutter. Furthermore, MIMO SDRs enable radar systems to process multiple channels in real-time, which is useful for multiple-antenna applications where different frequencies can be used to simultaneously cover different ranges or phased arrays can be used to focus the sensitivity using beam steering techniques. Finally, because these transceivers are modular, they can fit a variety of size, weight and power (SWaP) requirements, from large ground stations to small onboard drone units. Figure 2 illustrates a high level block diagram of a radar system with SDRs in the Rx/Tx chains for signal processing.
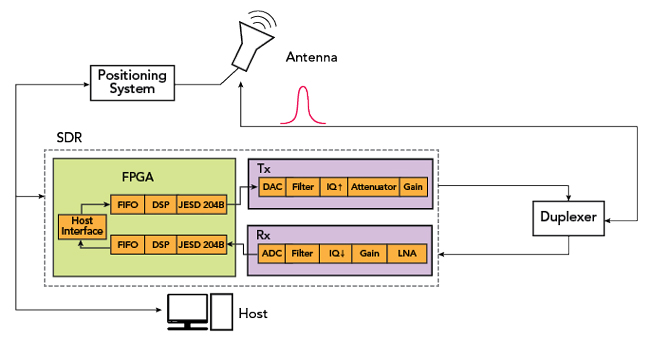
Figure 2 Representative radar block diagram with an SDR.
Spectrum monitoring and recording is another important RF application in aerospace and defense. This application uses powerful radio transceivers to detect and analyze the frequency content of the electromagnetic environment. SDRs are well-suited for spectrum monitoring systems since the RFE circuits have wide tuning ranges, high instantaneous bandwidth and several parallel channels with a low noise figure and high dynamic range, along with a high level of flexibility and reconfigurability. Spectrum monitoring systems using SDRs can provide real-time spectrum awareness, wideband signal monitoring and precise signal identification. This allows these systems to rapidly improve the spectrum sensitivity depending on the conditions of the RF environment. These systems use the flexibility and adaptability of the digital backend to monitor a wide range of frequencies and bandwidths simultaneously. This increases the sensitivity and selectivity of detecting and recording signals based on a programmed threshold that can be easily and remotely modified with software. MIMO SDRs can also significantly improve the spectrum coverage since each RF chain can cover a portion of the spectrum, independently, with a pre-defined gain/attenuation profile to optimize performance. High performance SDRs can provide high throughput backhaul for the host interface, allowing better integration with powerful storage solutions, along with incorporating network interface cards and NVMe drives to prevent data loss while optimizing consumption. Figure 3 shows a high level block diagram of a spectrum monitoring architecture and an SDR in the receive chain for signal processing and recording. In these systems, SDRs digitize and process the incoming signals, with sophisticated algorithms running on the backend for signal analysis and classification.
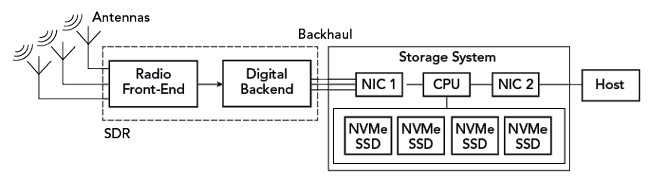
Figure 3 SDR integrated into a spectrum monitoring and recording solution.
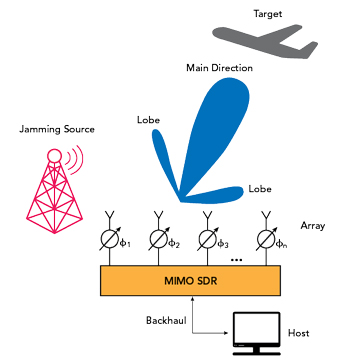
Figure 4 SDR and phased-array block diagram for EW applications.
EW is an important part of any modern military strategy and SDRs have become an essential tool for many EW applications, especially the ones involving aircraft and satellite operations. In these applications, SDRs perform electronic support measures (ESMs) to intercept and analyze electromagnetic signals coming from enemy radar and spectrum monitoring systems in real-time. These transceivers can also be used in electronic countermeasure (ECM) systems to jam or deceive enemy systems and in electronic attack (EA) systems to disrupt or destroy enemy devices. MIMO SDRs can augment EW transceiver capabilities by implementing ESM, ECM and EA operations simultaneously, using the dynamically controlled gain of the Tx and Rx chains to optimize signal detection and generation. SDR programmability enables rapid development and deployment of new EW capabilities as the threat landscape evolves, without the need for physical intervention or software replacement. The digital backend unit can rapidly adapt the modulation/demodulation scheme and communication protocol for advanced eavesdropping and anti-jamming techniques like frequency hopping. Figure 4 shows a block diagram of an EW transceiver that uses an SDR and beam steering to minimize the effects of jamming signals and adaptatively improve tracking sensitivity.
KEY AEROSPACE AND DEFENSE SDR SPECIFICATIONS
Selecting SDRs for aerospace and defense applications typically involves analyzing four critical performance indicators: tuning range, channel count, sampling bandwidth and digital backhaul. By analyzing these parameters, the engineer/designer can evaluate the device utility for a specific application, as well as how to optimize any possible limitations. The tuning range of an SDR establishes the frequency band where the RFE can receive and transmit signals with the proper gain and without major artifacts. In EW and spectrum monitoring applications, a wide tuning range is essential to quickly sweep between frequencies and optimize the probability of signal detection and monitoring. SDR-based radars can use their wide tuning range to direct different frequencies to multiple antennas to cover different requirements.
The channel count is also extremely important in aerospace and defense applications. Devices often need to acquire signals from completely different sources simultaneously, use phased array antenna systems for beamforming and perform multiple EW tasks in parallel as part of an “all-in-one” solution. In these cases, the MIMO SDR channels must be controlled, processed and activated independently to reduce latency and improve phase and frequency stability.
Sampling bandwidth is another frequency-related performance metric. The parameter defines the frequency range at which an SDR can sample accurately. This characteristic is essential to ensure sufficient frequency resolution to properly differentiate between harmonics for spectrum monitoring applications that capture and process high frequency, wide bandwidth signals in multiple channels.
Digital backhaul refers to the ability of an SDR to transmit and receive data over a digital interface that may be connected to a host or network. High speed digital backhaul is crucial for EW and spectrum monitoring applications. That connection enables rapid processing and analysis of large amounts of data with minimum loss of information.
It is important to note that other parameters may be more critical than these, depending on the exact device requirements. These parameters may include SWaP specifications, FPGA resources, FPGA accessibility, form factor and modularity level. Although some of these specifications are beyond the scope of the article, each SDR listed has benefits and drawbacks in these areas.
COMPARING SDRS
Having reviewed the basics of SDRs, the likely applications of these transceivers in aerospace and defense applications and important parameters to consider when selecting and designing these systems, it is time to compare the SDR models. This section compares SDRs from Per Vices, NI and Herrick Labs.
The Cyan SDR from Per Vices is a COTS transceiver targeting spectrum monitoring, radar and EW applications. This device offers a frequency tuning range from near DC to 18 GHz, making it an appropriate choice for high frequency applications. With a channel count accommodating up to 16 independent radio chains, this SDR can perform several different functions in the same device. This makes the device useful in applications like EW and spectrum monitoring, where multiple operations must be performed simultaneously and phased array radar systems with multiple antennas that require good phase and frequency coherence. The channel count, combined with 3 GHz instantaneous bandwidth and 24-bit/32-bit resolution per channel, increases the spectrum coverage capabilities of this device. Each channel can be assigned to a different portion of the spectrum with optimized gain and power thresholds.
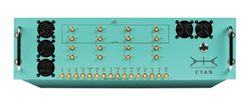
Figure 5 Cyan SDR from Per Vices.1
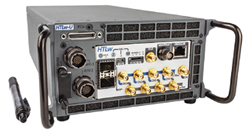
Figure 6 The HTLw SDR from Herrick Labs.2
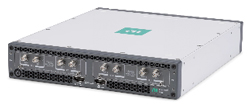
Figure 7 The USRP X410 from National Instruments.3
The backhaul architecture of this transceiver allows up to 4 × 40 Gbps, upgradable to 4 × 100 Gbps in the high bandwidth version, channels over optical qSFP+ links, enabling high data throughput and very low latency backhaul. This is a bottleneck for many SDR models and this feature satisfies a need in high performance recording solutions. The trade-off with these performance capabilities is that the Cyan SDR does not provide the best SWaP solution. Figure 5 shows the CYAN SDR.
The HTLw SDR, from Herrick Labs, can operate with a tuning range from 20 MHz to 18 GHz while providing an instantaneous bandwidth of about 1 GHz per channel. This enables the device to be used in a variety of high frequency applications, including spectrum monitoring, radars and complex EW systems. With four Tx and four Rx channels, it has a lower channel count than Cyan, but the advantage is a smaller form factor. One of the most significant benefits of the HTLw is the low phase noise per channel, combined with high bandwidth, making it suitable for many systems. It also features a powerful FPGA with onboard ARM9 cores and NEON coprocessors. The FPGA is capable of handling new, complex waveforms and heavy parallel signal processing in multiple channels. The HTLw provides two 10 GbE Ethernet ports with a compact design that makes it suitable for low SWaP onboard applications. This SDR is shown in Figure 6.
The final model is the NI USRP X410. This product is part of their universal software radio peripheral (USRP) line of products. This family of SDRs is open-source, allowing designers to create customized solutions. In this device, the digital backend is based on the Xilinx Zynq Ultrascale+ ZU28DR RFSoC, featuring a 12-bit resolution DAC and a 14-bit ADC that provides good computational resolution. The USRP RFE tuning range is 1 MHz to nearly 8 GHz, with up to 400 MHz of instantaneous bandwidth per channel. This is sufficient for applications including real-time signal processing and rapid prototyping, but insufficient for complex spectrum monitoring solutions. The SWaP characteristics of this transceiver are its differentiator. This device houses four Tx and four Rx channels in a half-wide RU form factor. This makes for a high channel density, but the total channel count is significantly lower than some of its competitors. This makes the X410 an attractive option for portable, onboard and battery-powered applications, thanks to its relatively low power consumption and small form factor. The backhaul has two QSFP28 ports going up to 10/100 GbE and a high speed data communication host interface that is not compromised by the small form factor. Although the USRP X410 is limited in terms of channel count and bandwidth when compared to the other two models, its flexibility and compatibility with popular software platforms, like LabVIEW and MATLAB, make this transceiver an excellent choice for engineers and researchers looking to prototype and test novel architectures for the aerospace industry. The USRP X410 is shown in Figure 7.
When selecting the most appropriate SDR for a particular application, several factors need to be considered. The number of channels, bandwidth, form factor and backhaul requirements all play a vital role in determining which SDR is best suited for a specific application. For instance, if a user requires a high number of channels, along with high bandwidth operation and high throughput backhaul, the Per Vices Cyan would be an excellent choice. However, it may not be the most suitable option for applications with strict SWaP requirements. On the other hand, the Herrick Labs HTLw provides high frequency performance with a small form factor and high spurious-free dynamic range, but at the cost of a lower channel count. Additionally, the NI USRP X410 is a budget-friendly option that offers great value for fast prototyping and non-critical applications, but it provides lower overall performance compared to the other two models. Ultimately, it is essential to understand the performance parameters and their application-specific benefits when selecting an SDR for a particular use case. A comparison of the key performance metrics of the referenced SDRs is shown in Table 1.
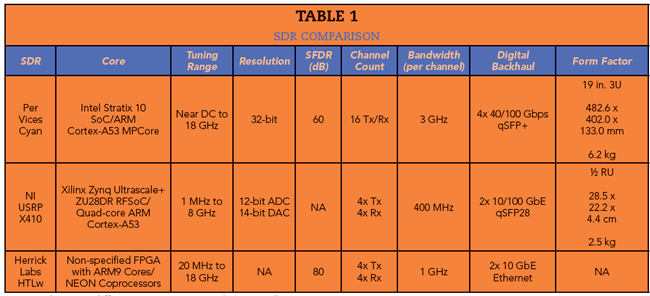
CONCLUSION
SDRs are becoming increasingly important in the aerospace and defense industries due to their ability to adapt to different use cases and protocols. Key specifications for SDRs in these markets include tuning range, channel count, sampling bandwidth and digital backhaul. The Per Vices Cyan Model, the NI USRP X410 and the Herrick Labs HTLw are examples of currently available, high performance SDRs with their own unique set of specifications and benefits. Overall, SDRs play a critical role in this industry, so the selection of the appropriate transceiver for a particular use case greatly impacts the performance and success of any mission and should not be taken for granted.
References
- Per Vices, Cyan, Web: www.pervices.com/cyan.
- Herrick Labs, HTL Products, Web: www.herricktechlabs.com/htl-products-and-solutions/htl-core-radios.
- NI, Ettus USRP X410, Web: www.ni.com/pt-pt/support/model.ettus-usrp-x410.html.