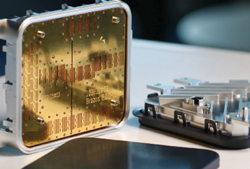
Figure 1 Automotive imaging radar antenna with housing.
The shift to mmWave frequency bands and the demand for more reliable sensors are putting ever-increasing pressure on automotive radar capabilities, challenging what classical printed circuit board (PCB) antennas can provide. Gapwaveguide technology has established itself as an attractive solution by offering excellent performance and advanced features at a competitive price (see Figure 1). This article gives an overview of the range of applications, solutions and relevant aspects of how this unique technology is enabling large and small players to make cost-effective high performance automotive radar a reality. An insight is given into the technical as well as the industrialization aspects.
Despite the technical challenges, the microwave industry has constantly reached out to higher frequencies. On the one hand, motivations are connected to necessities, such as the congestion of the already exploited spectrum, as well as to advantages, such as larger bandwidth and smaller form factors. On the other hand, the obstacles have always been the lower performance of the circuitry and less favorable propagating conditions. Recently, modern telecommunication and radar systems have moved well into the mmWave frequency bands. While this fits within this larger trend and evolution, it is a significant leap that presents considerable challenges. As a result, the technology is pushing an evolution to several established solutions in the industry, from signal processing to hardware.
When it comes to RF distribution and radiation, planar solutions based on PCB technology have often been the preferred choice, offering adequate performance for most applications at low cost with simple integration. This has also been the case for automotive radar in previous lower frequency bands and the first generations of the new 77 GHz band. However, at mmWave frequencies, issues such as low bandwidth, high losses and expensive RF substrates become significant and the perceived PCB limitations create opportunities for other technologies. Some of the alternative candidate technologies include traditional waveguides, substrate integrated waveguides (SIW), low temperature co-fired ceramics (LTCC) and lens antennas.
AUTOMOTIVE RADAR APPLICATIONS
Automotive radars have completed the migration to the 76 to 81 GHz band, now also mandated by international regulatory bodies.1 The main benefits are more accurate distance measurement due to the wider band and the easier integration of sensors on the car due to size reduction. Meanwhile, the increasing functionalities of advanced driver assistance systems (ADAS) and the rise of autonomous driving (AD) demand more capable and accurate sensors. Together with the other onboard sensors, such as cameras and lidars, radars are expected to continue having a significant role thanks to their low-cost and all-weather operation. However, to do so, radars must keep improving their performance while continuing to meet strict cost, size, thermal and reliability requirements.
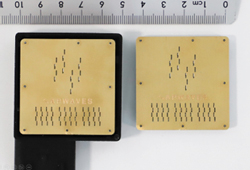
Figure 2 Compact and low cost corner radar antennas for ADAS.
To reach an accurate and complete perception of the vehicle’s surroundings, multiple radar sensor types exist.2 In ADAS applications, one often distinguishes between short-, mid- and long-range radars, based on the distance and field of view (FoV) needed for the corresponding functions, such as blind spot detection, lane change assist and forward collision warning, respectively. These sensors are specialized and increasingly ubiquitous, with massive volumes captured by a few large TIER-1 suppliers. They typically have between eight to 16 channels in a compact form factor and cost is a key factor (see Figure 2). The main performance challenge here is reaching the desired range and FoV. On the opposite end of the spectrum are the more powerful and flexible high-resolution sensors, used for example in AD systems. They are also referred to as imaging radars (see Figure 1) due to their ability to provide camera/lidar-like perception through high resolution. These more advanced and premium sensors, with 30 to 100 channels, target a young market with multiple players and smaller volumes. The main performance challenges are to realize low loss routing and complex large antennas with a massive number of channels. Clearly, the wide range of sensor types means a correspondingly broad range of priorities and optimal solutions that shall be addressed.
GAPWAVEGUIDE TECHNOLOGY
Traditional waveguides are one of the earliest and best performing RF technologies, but bulkiness and high cost have limited them to niche high-end applications, such as satellite and military. With the miniaturization at mmWave frequency bands, only cost remains as a major obstacle even more so since tolerances become increasingly stringent. In fact, one of the most challenging and costly manufacturing aspects is ensuring an accurate fit between parts. For this reason, high precision aluminum milling and many screws are typically used. More scalable solutions exist, such as dip brazing, soldering and 3D printing, however they remain expensive and technically demanding, so not suitable for mass production.
Meanwhile, gapwaveguide technology has risen as a solution to reach the same performance in a robust and cost-effective way.3 In the most typical implementation, an equivalent transmission line can be obtained simply by replacing the waveguide side walls with an artificial magnetic conductor (AMC) texture, typically in the form of periodic posts of appropriate dimensions. Then, even in the presence of mating defects in the manufacturing or assembly of the parts, the fields remain well confined in the waveguide channel, unlike traditional hollow waveguides which instead suffer a field breakdown, as shown in Figure 3. In theory, gapwaveguide technology works for gaps up to a quarter of wavelength, 0.9 mm (about 0.04 in) at E-Band, but it is used to relax tolerances and thus lower the cost.
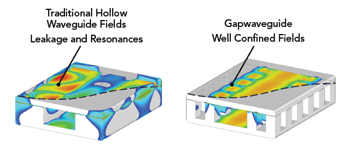
Figure 3 With small assembly defects, the fields in a standard waveguide can break down (left) while a gapwaveguide operates as designed (right).
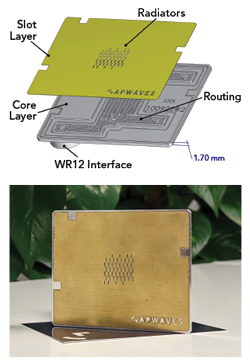
Figure 4 Gapwaves automotive reference antenna fabricated and assembled (bottom); exploded view (top).
GAPWAVES AUTOMOTIVE SOLUTIONS
Back in 2018, amid initial skepticism in the industry on waveguide solutions, Gapwaves released an automotive reference antenna,4 shown in Figure 4. Rather than an actual product, the purpose was to allow customers’ engineering teams a quick firsthand evaluation of some key capabilities through standard WR12 interfaces. From a form factor point of view, the design sported a minimalistic 1.5 layers (one core layer and a flat 2D metal one), sub 2 mm (about 0.08 in.) thickness and assembly by non-conductive glue. Performance-wise, the six channels supported the full 76 to 81 GHz band, half-wavelength spacing, narrow elevation coverage and wide and moderate in azimuth plane, using a series slotted waveguide end-fed design and optional grouping. Interestingly, despite being such an early demonstrator, several of these aspects have not yet been matched by competing technologies.