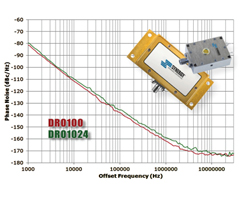
5G communication systems require high speed data transmission, extending the need for high frequency signal sources with low phase noise. Phase noise is an oscillator parameter that has grown in importance with the complexity of modern modulation formats, as phase noise can increase the bit error rate of a telecommunications link, degrade the stability of beams in particle accelerators and degrade the sensitivity of radar systems.
Responding to this need, Synergy Microwave has developed a line of compact, surface-mount, dielectric resonator oscillators (DROs) with extremely low phase noise at fundamental frequencies through 20 GHz.
ACHIEVING HIGH Q
Electronic oscillators generate low phase noise to a few GHz but the phase noise degrades at higher frequencies, principally due to low Q-factor resonators. Historically, quartz crystals were used as very high Q resonators, but their operating frequencies are limited to less than 200 MHz. The conventional approach to generating higher frequency signals is with a frequency multiplier, but this technique adds phase noise from AM-PM noise conversion and sub-harmonic generation.
Various resonators are used in electronic oscillators: printed coupled transmission lines using surface acoustic wave, dielectric, ceramic coaxial, YIG and sapphire-loaded cavity (SLC) resonators. All have unique characteristics and limitations. They typically operate from 500 MHz to 20 GHz; however, the Q degrades at higher frequencies, at best limited to f×Q < 1014. While SLC-based oscillators provide low phase noise signal generation, they have limited tuning capability and require precise low temperature cooling systems, which make them expensive.
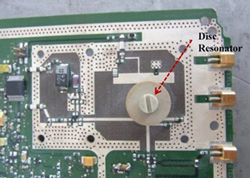
Figure 1 DRO circuit using a disk DR mounted on a PCB.
As an alternative, special composite ceramic structures called dielectric resonators (DRs) were developed to achieve a high Q resonator at microwave frequencies, subsequently leading to a family of oscillators called DROs. However, numerous challenges face the design and fabrication of low phase noise DROs at high frequencies, including the proper placement of the DR disk and coupling to the desired mode for stable oscillation. Figure 1 shows a typical DRO for understanding the DR placement on the printed circuit board. Typically, the DR is a disk attached through a spacer made of low dielectric material. Using a screw mounted on the DR, the frequency can be tuned across a narrow band. Unfortunately, DR placement using a spacer and puck for optimum coupling with a mechanical screw for tuning is sensitive to frequency drift under vibration and acceleration. For applications where g-sensitivity is important, this DRO construction has inherently poor phase noise performance.
METAMATERIAL-MÖBIUS STRIPS
With the aid of metamaterial-Möbius coupling, fundamental frequency DROs with low phase noise can operate through X- and K-Band, offering superior figures of merit (FOM).1 Manipulating and tailoring the electromagnetic wave coupling, several interesting properties of metamaterial-Möbius strips (MMS) can be achieved, such as reducing size for a given operating frequency and suppressing spurious resonance modes to improve the FOM of a tunable oscillator. The FOM can be a limiting factor in modern communications systems, especially those requiring a power efficient, low phase noise signal source. Möbius strips offer unique characteristics, including self-phase-injection properties along the mutually coupled surface of the strips, enhancing the Q for a given sized printed transmission line resonator. The Q is defined by:
where QL is loaded quality factor, φ (ω) is the phase of the oscillator’s open loop transfer function at a steady state and τd is the group delay of the MMS resonator.1
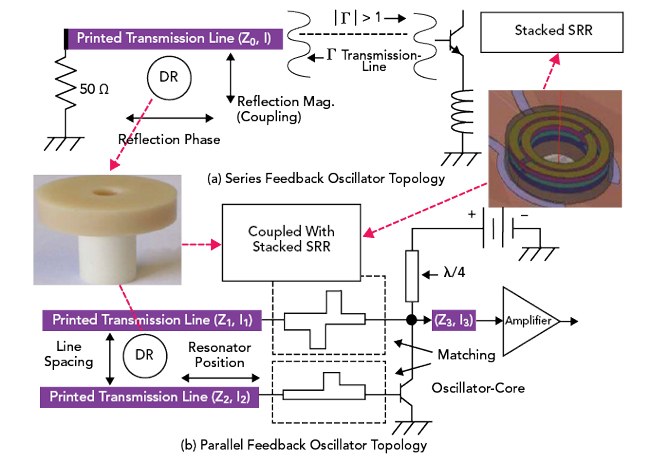
Figure 2 Typical DRO designs: reflection type with series feedback (a) and transmission type with parallel feedback (b).
MMS DRO PRODUCTS
Work on MMS DROs led Synergy Microwave to develop a line of compact, surface-mount DROs with extremely low phase noise at fundamental frequencies above 8 GHz, making them well suited for commercial, industrial and msupilitary applications. Figure 2 shows the typical architecture of a DRO using a disk and stacked split ring resonator (SRR) to realize a metamaterial-Möbius SRR frequency shaping resonant module. This yields an oscillator stable enough to use as a low phase noise local oscillator. Figure 3 shows a block diagram of a K-Band version. An X-Band metamaterial-Möbius DRO achieves a phase noise of -139 dBc/Hz at 10 kHz offset from a 10.24 GHz carrier (see Figure 4), which supports applications such as radar systems requiring high dynamic range and low radar cross section target detection. For systems requiring compact sources, Synergy offers metamaterial-Möbius DROs in both connectorized and surface-mount housings, measuring 0.75 × 0.75 in. The SMD versions can be extended to any number of fixed frequencies, typically from 8 to 20 GHz, without long lead times to produce the sources. These MMS DROs typically draw 30 mA and should be powered by a clean DC bias voltage, using an external regulator if necessary to minimize supply variation.
Figure 3 Mobius stacked SRR K-Band oscillator.
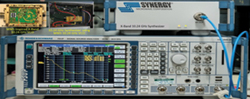
Figure 4 Phase noise measurement of a 10.24 GHz MMS DRO.
A standard MMS DRO product set for a 10 GHz output frequency can be mechanically adjusted by about ±50 MHz. To compensate for the frequency drift in phase-locked systems, an electrical tuning port enables ±1 MHz adjustment using a tuning range from +1 to +15 VDC. The operating temperature range is from -25°C to +70°C. By mode-locking, the operating temperature can be extended to -40°C to +85°C, providing excellent output power and phase noise performance over temperature.
The MMS DROs developed by Synergy Microwave are used widely in military and commercial applications, including wireless LAN and other communications systems, test and measurement, electronic warfare, missile, radar and medical.1,2
Synergy Microwave Corp.
Paterson, N.J.
References
- A. K. Poddar, “Slow Wave Resonator Based Tunable Multi-Band Multi-Mode Injection-Locked Oscillators,” Dr. In. Habil Thesis 2013, BTU Cottbus, Germany.
- A. S. Daryoush, A.K. Poddar, T. Sun and U. L. Rohde, “Optoelectronic Oscillators: Recent and Emerging Trends,” Microwave Journal, October 2018, pp. 58–76.