IMPLEMENTATION AND MEASUREMENT RESULTS
The dual-bias network is verified with a DPA designed for 5G mobile communications in the frequency band of 3.4 to 3.6 GHz. Figure 5 is a photograph of the dual-bias DPA. It is fabricated on a 30 mil thick Rogers RO4350B substrate with a 3.48 dielectric constant. Cree CGH40010F GaN HEMTs are used for both the carrier and peaking amplifiers. The carrier amplifier is set to Class AB with a gate bias of -2.75 V. The peaking amplifier operates in Class C with gate bias of -6 V. Referring to the transistor datasheet, the drain operating voltages of both amplifiers are set to 28 V.
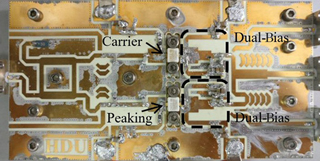
Figure 5 Fabricated GaN DPA.
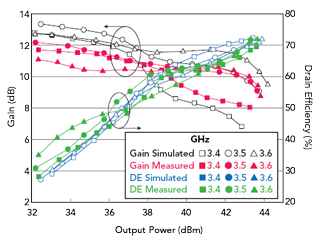
Figure 6 Measured and simulated gain and drain efficiency vs. output power, 3.4 to 3.6 GHz.
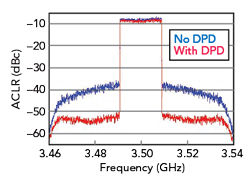
Figure 7 Measured ACLR at 3.5 GHz.
The curves of the gain and efficiency of the DPA as a function of output power are shown in Figure 6. Saturated output power can reach more than 43 dBm. Compared with the simulated efficiency, at low output power, the measured efficiency is higher and at higher output power, the measured efficiency is about three percentage points lower. The drain efficiency at saturation is above 70 percent. When the output power is backed off 6 dB, it is in the range of 51 to 55 percent. The efficiency is higher than 43 percent when the output power is backed off by 8 dB. Measured gain is slightly lower than simulated. When approaching saturated output power, gain appears to compress, but the average measured gain is greater than 10 dB.
At the 3.5 GHz center frequency a 20 MHz LTE modulation signal with a peak-to-average power ratio of 7.1 dB is used to drive the DPA. The measured ACLR is shown in Figure 7. Upper and lower sidebands are -32.1 and -31.9 dBc, respectively, with an output power of 42.7 dBm. After the addition of DPD they are -46.6 and -47.5 dBc, respectively.
Table 1 provides a performance comparison with other published DPAs, showing improved drain efficiency and ACLR.
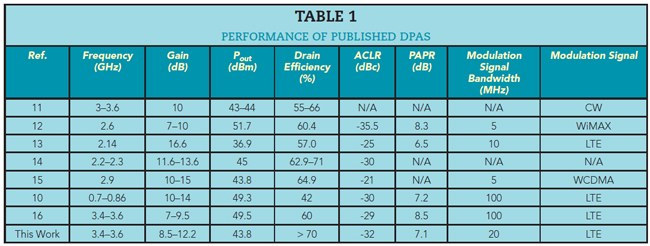
CONCLUSION
A new type of dual-bias network structure widens RFPA VBW and reduces the memory effect. To verify this, a DPA operating from 3.4 to 3.6 GHz is designed and fabricated. The ACLR measured with DPD is lower than -46 dBc when using a 20 MHz LTE modulation signal with a PAPR of 7.1 dB. This shows that the dual-bias structure not only widens the VBW and reduces the memory effect, but is also easily implemented.
ACKNOWLEDGMENTS
This work is supported by National Natural Science Foundation of China (No. 61871169).
References
- W. H. Doherty, “A New High Efficiency Power Amplifier for Modulated Waves,” Proceedings of the Institute of Radio Engineers, Vol. 24, No. 9, September 1936, pp. 1163–1182.
- R. Pengelly, C. Fager and M. Ozen, “Doherty’s Legacy: A History of the Doherty Power Amplifier from 1936 to the Present Day,” IEEE Microwave Magazine, Vol. 17, No. 2, February 2016, pp. 41–58.
- R. Darraji, D. Bhaskar, T. Sharma, M. Helaoui, P. Mousavi and F. M. Ghannouchi, “Generalized Theory and Design Methodology of Wideband Doherty Amplifiers Applied to the Realization of an Octave-Bandwidth Prototype,” IEEE Transactions on Microwave Theory and Techniques, Vol. 65, No. 8, August 2017, pp. 3014–3023.
- J. Wong, N. Watanabe and A. Grebennikov, “High-Power High-Efficiency Broadband GaN HEMT Doherty Amplifiers for Base Station Applications,” IEEE Topical Conference on RF/Microwave Power Amplifiers for Radio and Wireless Applications, January 2018.
- M. Franco, A. Guida, A. Katz and P. Herczfeld, “Minimization of Bias-Induced Memory Effects in UHF Radio Frequency High Power Amplifiers with Broadband Signals,” Proceedings of the IEEE Radio Wireless Symposium, January 2007, pp. 369–372.
- I. Takenaka, K. Ishikura, H. Takahashi, K. Hasegawa, K. Asano and N. Iwata, “Improvement of Intermodulation Distortion Asymmetry Characteristics with Wideband Microwave Signals in High Power Amplifiers,” IEEE Transactions on Microwave Theory and Techniques, Vol. 56, No. 6, June 2008, pp. 1355–1363.
- K. Moon, Y. Cho, J. Kim, S. Jin, B. Park, D. Kim and B. Kim, “Investigation of Intermodulation Distortion of Envelope Tracking Power Amplifier for Linearity Improvement,” IEEE Transactions on Microwave Theory and Techniques, Vol. 63, No. 4, April 2015, pp. 1324–1333.
- Y. Cho, D. Kang, K. Moon, D. Jeong and B. Kim, “A Handy Dandy Doherty PA: A Linear Doherty Power Amplifier for Mobile Handset Application,” IEEE Microwave Magazine, Vol. 18, No. 6, September-October 2017, pp. 110–124.
- H. H. Ladhani, J. K. Jones and G. Bouisse, “Improvements in the Instantaneous-Bandwidth Capability of RF Power Transistors Using in-Package High-k Capacitors,” IEEE MTT-S International Microwave Symposium, June 2011.
- C. Ma, W. Pan, S. Shao, C. Qing and Y. Tang, “A Wideband Doherty Power Amplifier with 100 MHz Instantaneous Bandwidth for LTE-Advanced Applications,” IEEE Microwave and Wireless Components Letters, Vol. 23, No. 11, November 2013, pp. 614–616
- J. M. Rubio, J. Fang, V. Camarchia, R. Quaglia, M. Pirola and G. Ghione, “3-3.6 GHz Wideband GaN Doherty Power Amplifier Exploiting Output Compensation Stages,” IEEE Transactions on Microwave Theory and Techniques, Vol. 60, No. 8, August 2012, pp. 2543–2548.
- J. Son, I. Kim, J. Moon, J. Lee and B. Kim, “A Highly Efficient Asymmetric Doherty Power Amplifier with a New Output Combining Circuit,” Proceedings of the IEEE International Conference on Microwaves, Communications, Antennas and Electronic Systems, November 2011.
- H. Oh, H. Kang, H. Lee, H. Koo, M. Kim, W. Lee, W. Lim, C. S. Park, K. C. Hwang, K. Y. Lee and Y. Yang, “Doherty Power Amplifier Based on the Fundamental Current Ratio for Asymmetric Cells,” IEEE Transactions on Microwave Theory and Techniques, Vol. 65, No. 11, November 2017, pp. 4190–4197.
- W. Shi, S. He and N. Gideon, “Extending High-Efficiency Power Range of Symmetrical Doherty Power Amplifiers by Taking Advantage of Peaking Stage,” IET Microwaves, Antennas & Propagation, Vol. 11, No. 9, July 2017, pp. 1296–1302.
- Y. Peng, L. Zhang, J. Fu, Y. Wang and Y. Len, “Modified Output Impedance Matching Solution for Load Modulation Power Amplifier Performance Enhancing,” IET Microwaves, Antennas & Propagation, Vol. 9, No. 13, October 2015, pp. 1376–1385.
- J. Xia, X. Zhu, L. Zhang, J. Zhai and Y. Sun, “High-Efficiency GaN Doherty Power Amplifier for 100-MHz LTE-Advanced Application Based on Modified Load Modulation Network,” IEEE Transactions on Microwave Theory and Techniques, Vol. 61, No. 8, August 2013, pp. 2911–2921.
- J. Brinkhoff, A.E. Parker and M. Leung, “Baseband Impedance and Linearization of FET Circuits,” IEEE Transactions on Microwave Theory and Techniques, Vol. 51, No. 12, December 2003, pp. 2523–2530.
- Y. Cho, D. Kang, J. Kim, K. Moon, B. Park and B. Kim, “Linear Doherty Power Amplifier with an Enhanced Back-Off Efficiency Mode for Handset Applications,” IEEE Transactions on Microwave Theory and Techniques, Vol. 62, No. 3, March 2014, pp. 567–578.