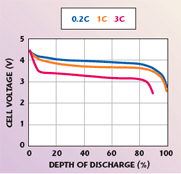
Next-generation mobile phones are capable of downloading music and video programming, displaying global positioning system (GPS) mapping and sharing high resolution photographs. These new applications are becoming increasingly popular as teenagers and others learn to manipulate photo and music files. Applications involving heavy display usage (such as mobile television) will significantly affect the overall power budget in a mobile phone. As consumers evolve their phone usage habits to include these more data-intensive applications, total battery storage requirements will dramatically change. Battery life reserved for handset talk time is no longer sufficient.

Battery Technology
In order to stretch battery technology to permit higher energy density and longer battery life, the world’s leading battery suppliers are looking at alternative chemistry and fabrication techniques which will result in lower discharge voltages. Today’s mobile phones are designed to use batteries at discharge voltages above 3.0 to 3.5 V, where lithium-ion batteries have an excellent discharge characteristic. Figure 1 illustrates the voltage profile for a variety of discharge conditions. (1C refers to the current draw required to drain the nominal battery capacity in one hour; for example, for a 650 mAh battery the current level at 1C would be 650 mA.) Note that as a battery is drained more quickly, its capacity is reduced. At 3C the lithium-ion battery is effectively depleted at 90 percent of its rated capacity. The point at which the voltage drops off near complete discharge is often referred to as the “cut-off voltage.”

In particular, battery suppliers have developed alternative cathode and anode materials that improve volumetric energy density (energy per liter) or gravimetric energy density (energy per kilogram or specific energy) of a cell. Lithium-ion cells are currently best for size-critical applications such as mobile phones, so much of the recent development focus has been on the addition of new metals for additional cathode chemistry complexity. By substituting manganese or nickel in the cathode, volumetric density can be dramatically increased, but at the expense of discharge voltage. This has been followed up by the substitution of silicon for the graphite (carbon) anode in order to extend the performance of the cell as much as possible. The anode change causes the discharge characteristic to slope even more, resulting in a more dramatic decline in available voltage and a more dramatic leap forward in volumetric energy density. Figure 2 and Table 1 describe the impact: By giving up as much as 0.6 V of discharge voltage, the new batteries can improve energy density by as much as 40 percent.
Note: another alternative to lithium-ion batteries, especially for weight-critical applications, is lithium sulfur cell technology. Lithium sulfur batteries, since they use no heavy metals, are lighter and more environmentally friendly than lithium-ion batteries. With its liquid cathode construction, the lithium sulfur system can deliver high current readily and can reportedly reach gravimetric densities of over 300 Wh/kg and volumetric density of roughly 450 Wh/liter. Lithium sulfur cells currently operate nominally at 2.1 V, with a cut-off voltage at less than 2.0 V. The “green” nature of this approach makes lithium sulfur a possible candidate for high volume applications such as mobile phones.

The Problem
Why haven’t mobile phones already taken advantage of the benefits that are possible with changes to the battery? The limitation comes down to a short list of components that have not been scrutinized for their ability to cope with lower voltage. In particular, many system designers believe that RF power amplifiers need to be supplied with 3 V or higher, due to the heavy use of HBT amplifier technology in the marketplace at present.
Today, the majority of the mobile handset market uses heterojunction bipolar transistors (HBT) for the RF power amplifiers in handsets. For HBT amplifiers, a forward bias is applied to the emitter-base junction, and a reverse bias is applied to the base-collector junction. The sandwich structure of the HBT device requires an overall voltage supply of 3.0 V to cause the device to “turn on” for high power amplification (at low RF power levels, the bias voltage can be a bit lower with acceptable degradation in linearity). As the market considers the use of low voltage battery cells, some HBT amplifier users are now considering the addition of a buck/boost DC/DC voltage converter in order to maintain adequate bias voltage. This would obviously add cost and reduce efficiency of the overall amplifier solution.

Complexity with Bias Control Schemes
DC/DC converters are often used in the design of mobile handsets today, stepping down the bias voltage for a power amplifier during transmissions that only require low RF power output. Figure 3 illustrates the benefit derived from adjusting the bias voltage to be lower in the case of both E-pHEMT and HBT amplifiers. For optimal efficiency at all power levels, the voltage was adjusted continuously according to a preset profile. Note that in both cases, the same bias voltage profile was used, demonstrating that the E-pHEMT amplifier inherently performs at higher efficiency than its HBT counterpart.
Amplifiers are also often switched into different states in order to save power. Many amplifiers have been introduced to the market in which the output section may be switched out of the signal path, allowing the driver section of the amplifier to supply the output power at much higher power-added efficiency (PAE). The most efficient of these designs make use of a patented “switchless” technology developed by Avago Technologies.
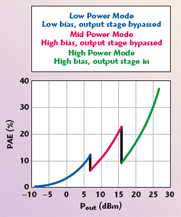
Figure 4 shows the impact of switched amplifiers. In this case, a “switchless” architecture was used, meaning that the bias voltage was simply set to zero for the bypass condition, with a specialized matching network that enabled a low loss bypass network to direct the signal from the driver stage to the antenna port. To simplify the demonstration, in the mid-power and high power ranges the bias voltage was simply set to 3.4 V, while at low power the bias was set to a lower constant voltage. In the end, a combination of continuously variable bias voltage and switched amplifiers can realize a power amplifier for W-CDMA or CDMA operation with a PAE in the 20 to 40 percent range throughout the entire dynamic operating range of the system.
The Solution
In contrast with HBTs, FET devices operate on a different principle, allowing them to operate at much lower bias voltages. E-pHEMT (enhancement mode pseudomorphic high electron mobility transistor) technology offers an excellent alternative. With the highest efficiency in the industry at high voltages, E-pHEMT devices become even more compelling at low bias voltages. Because E-pHEMT devices can maintain linearity and gain at low bias voltages, below 2 V, the cost and electrical loss in the buck/boost converter can be avoided. Efficiency and handset talk time can be maximized while avoiding unnecessary components.

Figure 5 illustrates the point that E-pHEMT amplifiers can maintain predictable and useful performance as low as 1.5 V, and possibly lower. In the plot, a standard E-pHEMT amplifier was subjected to a variety of bias conditions using a standard load matching network and test waveform. At extremely low voltage (0.4 V), engineers were able to re-tune the load matching network in order to re-optimize the amplifier’s performance and achieve a level of 20 dB gain for low power levels.
Many handset manufacturers already adjust the output power of the RFIC to compensate for changes in amplifier gain at varying bias voltages. Often this is done at the low power or mid-power transmitter levels, where linearity performance is not critical for the amplifier. In other words, when the mobile handset is fairly close to the base station (within a mile or so), the transmitter power from the handset can be backed off. This relaxes the performance requirements for the amplifier in terms of intermodulation distortion and other forms of nonlinear behavior. However, in the case of a new battery cell technology, the voltage will be set at a lower level for high output power levels as well, creating a more critical need for high linearity performance up to 24 dBm output power and higher. Note that the actual output power required from the amplifier varies depending on the output duplexer or switch technologies chosen; bulk acoustic wave (BAW) filters such as film bulk acoustic resonator (FBAR) filters can reduce the output loss, reducing the amplifier’s output power rating. A combination of E-pHEMT amplifiers and low loss filters can address the requirements for high antenna output power and high linearity simultaneously with low supply voltage.
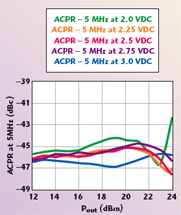
Figure 6 demonstrates the linear performance of the E-pHEMT amplifier over a wide range of bias voltage conditions. Typical specifications call for –35 dBc adjacent channel power ratio (ACPR) performance for a W-CDMA signal. The E-pHEMT amplifier easily exceeds this requirement, with margin for mass production up to a transmitter power of 24 dBm. In fact, below 24 dBm the impact of the bias voltage on linearity is not measurable by a typical test set. Only at high power does the linearity degrade slightly as the bias voltage is turned down to 2.0 V. Based on these simple tests, it is clear that E-pHEMT technology can maintain high linearity and flat, predictable AM/AM and AM/PM performance at low bias levels.
Dozens of innovative ideas have certainly been employed to squeeze the maximum linear RF power out of the smallest possible amount of DC power. As a new generation of mobile phones is born with lower battery cell voltage, RF power amplifiers must also change directions. The end result will be incredible levels of efficiency and autonomous operation time for a typical handset. With new battery and PA techniques, consumers will be able to talk on their mobile phones for extended periods and still enjoy the latest offerings in multimedia content. n

Joe Madden
Joe Madden holds a BS degree in physics from UCLA. He is responsible for corporate business development at Avago Technologies, a spinout of Agilent Technologies Inc. He has been involved in wireless product marketing for over fifteen years, with a few years in the lab designing filters and other RF devices. Before joining Avago, he served as president of STI-Sunnyvale and as vice president of marketing at Spectrian Corp. He has published papers ranging from molecular-beam epitaxy to digital amplifier predistortion.